water level & depth
more information....
borehole and groundwater monitoring
Water level measurements in borehole monitoring wells (Figure 1) are undertaken for groundwater and aquifer monitoring. Water level sensors quantify the level of the water table, or groundwater table, in a landscape or aquifer. Where water level fluctuates, water level sensors can quantify the groundwater zone (saturated zone) which is separate to the vadose zone (unsaturated zone, Figure 2).
Water level sensors are also used in hydrology to quantify the potentiometric head across aquifers or landscapes. Potentiometric head, also known as potentiometric surface or piezometric surface, is the theoretical or imaginary surface to which water will rise in a confined aquifer if it was entirely exposed to borehole wells.
Water levels in boreholes and aquifers recharge due to precipitation (rainfall) events and subsequent vadose zone drainage. The size of the rainfall event determines the size of groundwater recharge. Water level sensors quantify the rate of recharge and determine how, or if, groundwater resources should be utilised.
Decreases in water level occur due to further drainage across the aquifer or groundwater system. Water level may also decline via transpiration from deep-rooted vegetation. The distribution and biogeography of certain vegetation types, known as groundwater dependent ecosystems (GDE’s) depend on access to groundwater and can influence water levels.
Therefore, an understanding of water level dynamics must also consider water inputs and outputs from the groundwater system. Consequently, water levels sensors are routinely used in combination with additional sensors such as a rain gauge (precipitation/rainfall), soil water content sensor (vadose zone dynamics), lysimeter (drainage), sap flow sensor (transpiration), and weather station (evapotranspiration and rainfall).
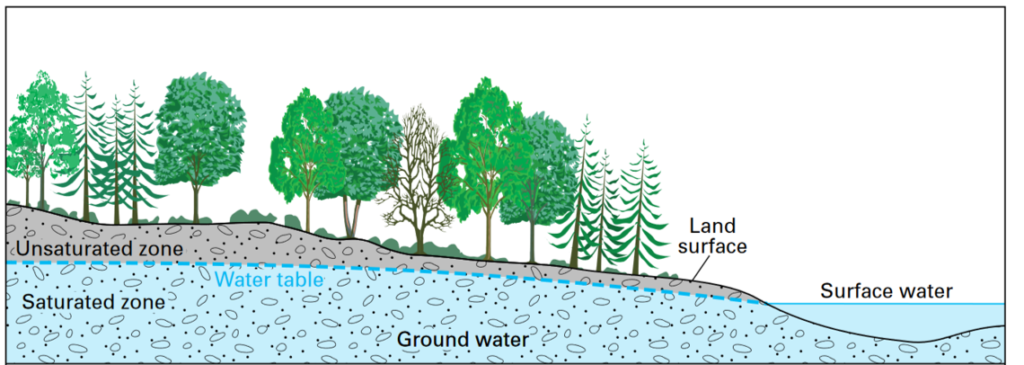
Figure 2. A depiction of and idealised landscape showing the distribution of groundwater and surface water. Source: USGS.
wireless sensor networks and internet enabled devices
An increasingly common application for water level monitoring is the integration of sensors into wireless sensor networks or internet enabled devices such as LoRa WAN, Sigfox, NB-IoT, and others. The water level sensors supplied by Edaphic Scientific, including the Implexx Water Level Sensor and HYDROS 21 Conductivity, Temperature and Depth (CTD) Sensor, are designed to easily integrate with wireless sensor networks and internet enabled devices. The sensors are low cost, require low power, have analog or digital output, and require low ongoing maintenance.
The SMART AGROWETLANDS II is an Italian based project that has integrated the HYDROS 21 (CTD-10) and TEROS 12 (GS3) digital sensors into a wireless sensor network (Figure 3). The project is monitoring soil water, groundwater, borehole, canal and irrigation water levels, alongside water quality parameters such as salinity (electrical conductivity) where sensors are connected to various nodes that send data to gateways to be accessed over the internet. The wireless sensor network is based on IEEE standard 802.15.4, which focuses on a low-cost and low-speed communication between nearby devices with little to no underlying infrastructure, and lower power consumption. The aim of the project is, by monitoring of soil, groundwater and channel water parameters, to formulate irrigation recommendations to support the farmers’ decisions across a sensitive hydrological landscape.

Figure 3. The distribution of sensor nodes in a wireless sensor network for the Agrowetlands Project. Source: Agrowetlands.
types of water level sensors
pressure transducer water level sensor
There is a direct relationship between water depth and pressure which can be used in water level sensors to determine water level. A pressure transducer water level sensor measures the pressure applied by the water column above the sensor.
Pressure transducer sensors measure water depth which can be converted to water level once a reference point, such as land surface, is known. A pressure transducer water level sensor must be referenced to atmospheric pressure. Some models of water level sensor require a surface based barometric pressure sensor and an offset equation in a data logger for accurate water depth measurements. Other models are “vented” where atmospheric air can enter the sensor, usually via a tube within the cable of the sensor, to correct for atmospheric pressure.
The Implexx Water Level Sensor and HYDROS 21 (Figure 4), available from Edaphic Scientific, are vented models of water level or depth sensors. A porous Teflon vent near the data logger end of the cable provides the reference (Figure 5). The Teflon keeps liquid water out of the cable but allows air to enter and leave. This vented apparatus allows for accurate measurements of water depth.
hydrostatic water level sensor
Similar to a pressure transducer water level sensor, a hydrostatic water level sensor measures the pressure of a liquid at a given depth. A hydrostatic sensor measures water depth which can be converted to water level once a reference point, such as land surface, is known. The equation for quantifying depth with a hydrostatic water level sensor is:
D = P/RG
where D is water depth, P is water or liquid pressure, R is the density of the liquid (where the density of water is approximately 1000 kg/m³ but varies with temperature), and G is gravity (9.8 m/s²).
piezometer or piezometric water level sensor
A piezometer is similar to the pressure transducer and hydrostatic style water level sensors. Often, the terms piezometric, hydrostatic and pressure transducer are used interchangeably to describe a sensor that measures the pressure head of water of liquid.
Piezometer is also used to describe other devices or mechanisms to measure water depth or water level. For example, a crude, or simple, piezometer may consist of a pipe, such as PVC, that is perforated at the borehole end that allows water level to increase and decrease within the pipe. Water level is then measured with a water level sensor or other device.
A vibrating wire piezometer is a more technologically sophisticated piezometric sensor. A vibrating wire piezometer consists of a magnetic, high tensile strength stretched wire, one end of which is anchored, and the other end is fixed, to a diaphragm. The frequency of vibration in the wire is calibrated against water pressure or water depth.
ultrasonic water level sensor
An ultrasonic water level sensor (Figure 6) is fundamentally different to a pressure transducer, hydrostatic or piezometric water level sensors as it is located above the body of water. An ultrasonic sensor emits an ultrasonic wave that is reflected from the water body back to the sensor’s receiver. The time lapse between when the ultrasonic wave is emitted and received by the sensor is related to distance (i.e. distance equals speed multiplied by time).
An ultrasonic water level sensor is more appropriate for applications where the sensor can be easily, and without obstruction, installed above a body of water such as tanks, dams, lakes, and rivers. An ultrasonic water level sensor may not be suitable for boreholes or wells because of the narrow diameter and long length of the access tube. For these applications, a pressure transducer water level sensor is more appropriate.
dipmeters for water level
A dipmeter, such as the Implexx Dipmeter (Figure 7), is a manual, portable device to measure the water level and groundwater of wells and boreholes. A dipmeter consists of a heavy stainless-steel probe at the end of a long, graduated tape.
The stainless-steel probe is lowered down a borehole or well and internal sensors indicate when it contacts water. The graduated tape, or LCD display on the dipmeter, indicates the water level.
A dipmeter is a useful and cost-effective device when there are numerous boreholes across a landscape. A dipmeter is a manual measurement, however, therefore it cannot be connected to a data logger for continuous readings.
A dipmeter provides excellent spatial resolution, but poor temporal resolution, of groundwater level dynamics across a landscape and aquifer.
water level scientific research
the effects of grasslands and forests on water level and groundwater
Mujica and Bea (2020) quantified plant available water (PAW), soil moisture, and water level dynamics with a range of sensors, including the HYDROS 21 (CTD-10), in landscapes consisting of grasslands versus forests. Salinity and salinization were also quantified with the sensors. The results showed that water level recharge was lower under forests than grassland because of more evapotranspiration from forest trees (Figure 8). These patterns also affected salinity levels across the landscapes.
Reference:
Mujica and Bea, 2020, Estimations of rooting depths and sources of plant-available water (PAW) in flatland petrocalcic soils under different land uses. Geoderma, 361, 114019. https://doi.org/10.1016/j.geoderma.2019.114019
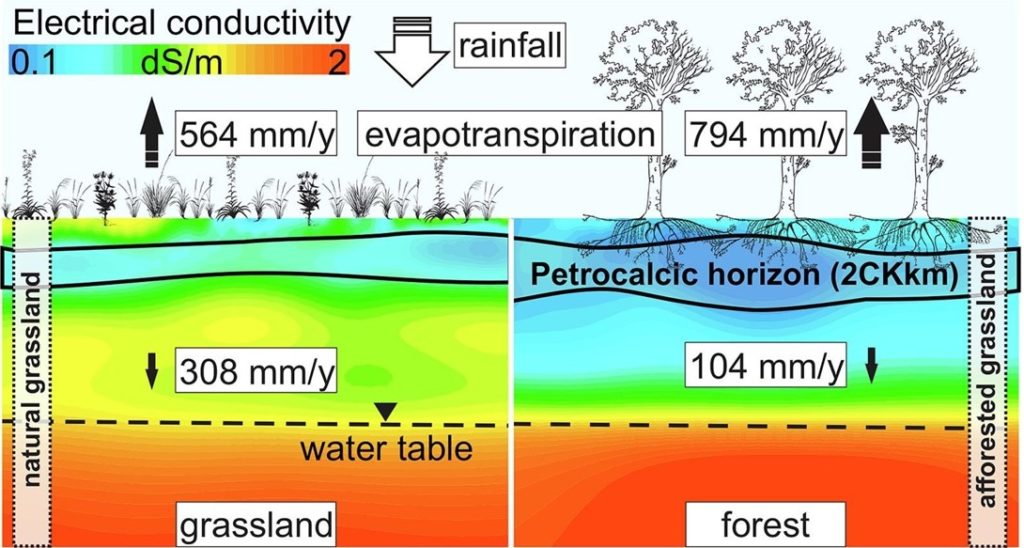
Figure 8. The effects of grassland and forests on soil salinity as measured with a HYDROS 21 CTD sensor. Source: Mujica and Bea (2020)
coastal wetland recharge and discharge dynamics
Coastal wetlands are recharged with freshwater from inland sources of water and precipitation (rainfall) as well as saline seawater. The relative rates and sources of recharge can significantly alter ecosystem function, and biodiversity, of wetlands. For example, rainfall can be highly seasonal, such as dry/wet seasons in the tropics or Mediterranean biomes. Manzoni et al (2020) quantified the seasonal water level dynamics of a Mediterranean wetland over 2 years. In this ecosystem, rainfall predominantly falls during the winter. The researchers measured water level with a HYDROS 21 (CTD-10) water level sensor as well as weather parameters such as rainfall. Figure 9 shows two years of rainfall, water temperature, water depth, and water EC (electrical conductivity) dynamics. Salinity in the wetland decreased following larger rainfall events. The authors concluded that “under future drier and warmer conditions, salinity in the lagoon is expected to increase, unless freshwater inputs are enhanced by restoring hydrologic connectivity between the lagoon and the surrounding freshwater bodies.”
Reference:
Manzoni et al, 2020, Understanding coastal wetland conditions and futures by closing their hydrologic balance: the case of the Gialova lagoon, Greece. Hydrology and Earth System Science, 24, 3557–3571. https://doi.org/10.5194/hess-24-3557-2020
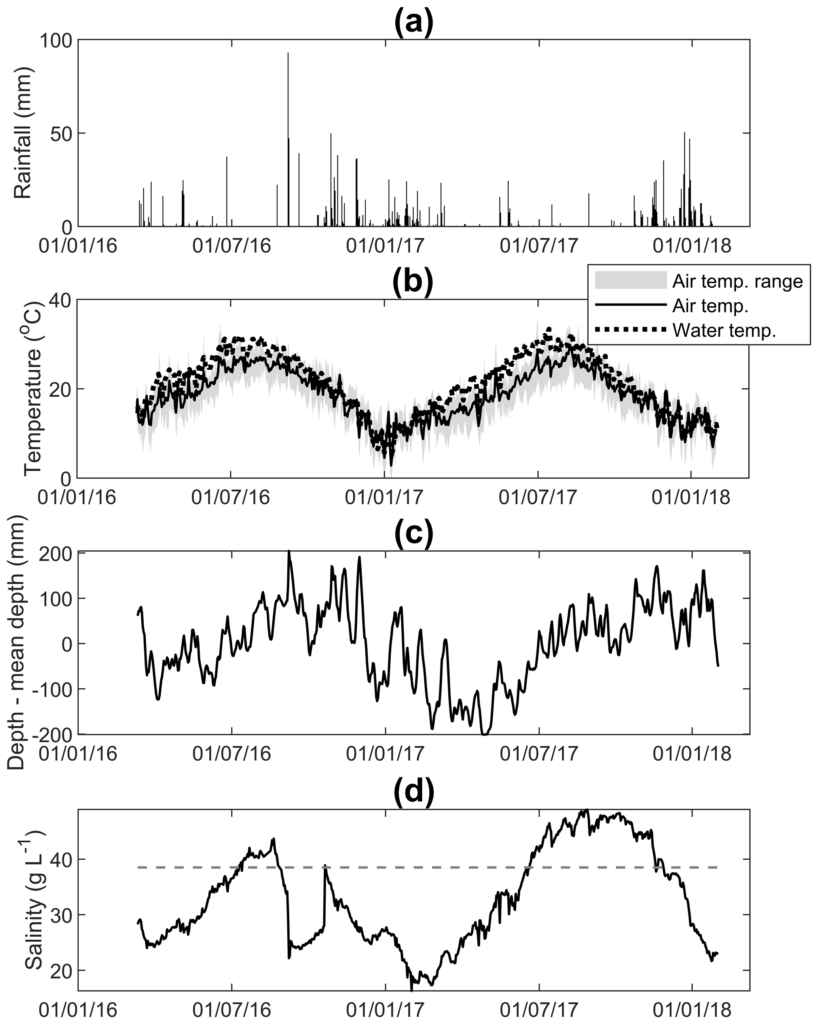
Figure 9. Two years of water level, temperature and EC measurements from a HYDROS 21 sensor. Source: Manzoni et al (2020).
additional information
- Reducing urban water pollution with water level sensors
- The Amerigo Lander: monitoring climate impacts in the oceans
- Aquaponics and water level sensors